10 Clean Air
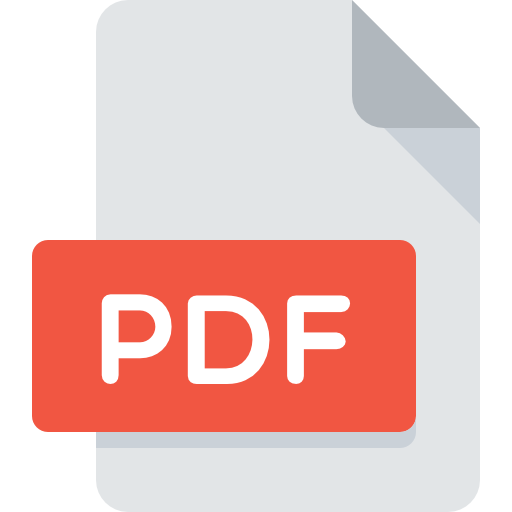
Improving and protecting human health is a key part of a clean growth transition. Health and climate outcomes are linked by the emissions we release into the atmosphere: some are greenhouse gas emissions and contribute to climate change, while others are air pollutants that are harmful to human health. In many cases, these air pollutants and GHG emissions are emitted at the same time from the same sources. As a result, Canada has significant opportunities to improve health as it accelerates action to reduce GHG emissions.
Headline Indicator #10: Ambient Air Quality across Canadian Cities
To measure air quality in Canada, we consider ambient air quality data in several Canadian cities across four major air pollutants (Figure 10.1). For comparison, we also include the Canadian Ambient Air Quality Standards (CAAQS) for 2020 and 2025, which are the baseline standards for air quality in Canada (see Box 10.1). Although we only have data for 2017–18 (see Data Gap section), the goal is to see ambient air quality improve over time.
The data in Figure 10.1 highlight a few notable trends. Overall, most Canadian cities in the figure achieved the 2020 and 2025 CAAQS in 2017–18, with a few exceptions. Vancouver was the only city in the figure that failed to meet the 2020 CAAQS for nitrogen dioxide; however, NO2 levels in Edmonton and Toronto exceeded the 2025 CAAQS. Two cities failed to meet the 2020 CAAQS for fine particulate matter (Edmonton, Saskatoon). None of the listed cities exceeded the CAAQS for ground-level ozone (smog), but many came close to the standard. As CAAQS continue to tighten over time, many towns and cities across the country (including many not in Figure 10.1) will need to improve ambient air quality to meet the national standards.
Box 10.1: Ambient Air Quality Standards in Canada
Ambient air quality refers to the concentration of pollution within a given airshed and changes based on the types and quantities of pollutants released into the local atmosphere. While pollution from human activities is the primary driver of poor air quality, weather conditions (wind, temperature, precipitation, etc.) and natural events (wildfires, volcanic eruptions) can also affect air quality.
To improve ambient air quality in Canada, the Canadian Ambient Air Quality Standards were developed collaboratively between federal, provincial, and territorial governments. Although there is no safe level of air pollution, the CAAQS establish baseline air quality standards for Canada that tighten every five years.
Source: CCME (2017).
The figure also highlights major differences in air quality across cities. Hamilton, for example, had among the highest concentration of sulphur dioxide (SO2) emissions in the country (concentrations were higher only in Trail, B.C., and Saguenay, Quebec). Industrial activity—and smelters in particular—are the largest source of SO2 emissions in Ontario and likely contributed to these high levels in Hamilton (Government of Ontario, 2017). Levels of fine particulate matter were nearly twice as high in Saskatoon and Edmonton compared to St. John’s and Halifax, which were likely driven by differences in wildfire activity and heavy industry.
Air quality can also be dramatically different within a city, as illustrated by the concentration of nitrogen dioxide (NO2) in Vancouver, which was twice as high as many other cities. One of Vancouver’s two monitoring stations is located on a busy stop-and-go trucking corridor (Clark/Knight Street) that serves Canada’s busiest port. Concentrations of NO2 at this station were nearly twice as high as levels in downtown Vancouver (located only a few kilometres away), pulling up the city’s average. In these urban driving conditions, heavy-duty trucks can emit NO2 emissions equivalent to 100 cars (Badshah et al., 2019).
Air pollution is not just a big-city problem. Ozone levels in Whitehorse were the fourth highest out of the 15 cities surveyed. Small and rural communities in southeastern and northeastern British Columbia had the highest levels of fine particulate matter in the country in 2017–18.
Lastly, the data in Figure 10.1 show that air pollution is not just a big-city problem. Ozone levels in Whitehorse, for example, were the fourth highest out of the 15 cities surveyed. Similarly, small and rural communities in southeastern and northeastern British Columbia have the highest levels of fine particulate matter (PM) in the country for 2017–18 (not included in the figure). Heavy wildfires during these two years were likely the primary cause of these high PM emissions, although emissions from forestry and resource extraction industries may have also contributed to poor air quality. Many Indigenous communities also grapple with poor air quality due to their proximity to industrial facilities and a heavy reliance on diesel generators in remote communities (MacDonald, 2019; CIRNAC, 2012).
Although Canadians generally enjoy relatively clean air compared to other countries, the ambient levels of air pollution presented in Figure 10.1 pose significant health risks, even in communities that achieve official air quality standards. Short-term exposure to air pollution—even at low levels—can cause shortness of breath, coughing, and chest pain. These health risks increase over time with longer and more regular exposure, increasing the risk of cancer, lung disease, irregular heart functions, cardiovascular disease, and even premature death (Health Canada, 2019; Wang et al., 2018; OECD, 2014).
Air pollution can also make existing problems worse. Emerging evidence from the COVID-19 global pandemic in 2020, for example, suggests that higher levels of air pollution increased the severity of the illness (Wu et al., 2020). Higher levels of air pollution can also exacerbate effects from climate change (Box 10.2).
In many cases, these health impacts are most acute in populations with other risk factors, such as children, seniors, and those with underlying health conditions. In children, for example, exposure to air pollution can increase the risk of respiratory issues and adversely affect cognitive development, such as weakened intelligence, memory, and behaviour capabilities (Heissel et al., 2019). Evidence also suggests some air pollutants (e.g., black carbon, particulates) can negatively affect prenatal development, leading to higher risk of autism, developmental delays, reduced IQ, anxiety, depression, ADHD, and reduced brain size (Bove et al., 2019; Payne-Sturges et al., 2018; Lancet Neurology, 2018; Fu et al., 2018; de Prado Bert et al., 2018). In elderly populations, air pollution is linked to respiratory issues and a higher risk of dementia, Parkinson’s disease, and multiple sclerosis (Sunyer et al., 2015; Chen et al., 2017).
Box 10.2: A Changing Climate Will Exacerbate Air Pollution Challenges
Air quality typically deteriorates in warmer temperatures. Concentrations of ground-level ozone, for example—a big component of smog—intensify in strong sunlight and heat. As global temperatures increase, the number of days with dangerously high levels of air pollution in Canada could increase, undoing some of the gains made over the past few decades in lowering air pollutant emissions.
Higher background levels of air pollution from buildings, transportation, and industry also worsen the health impacts from wildfire smoke. During the summer of 2018, for example, intense wildfire smoke over Metro Vancouver caused air quality to plummet, exposing over 1 million residents to a record-setting 22 days of air quality advisories. The wildfire smoke coincided with hot and dry summer conditions and was likely exacerbated by background levels of air pollution from buildings, transportation, and industry. As the risk of wildfires in Canada increases with worsening climate change, exposure to poor air quality also increases.
Sources: Metro Vancouver (2019); Lancet Countdown & Canadian Public Health Association (2017); Reid et al. (2016).
Figure 10.1 helps illustrate that a large portion of the Canadian population is regularly exposed to harmful levels of air pollution (Health Canada, 2017; Health Canada 2019; CCME, 2017). Over 70 per cent of Canada’s population lives in urban areas where concentrations of air pollutants are highest (Statistics Canada, 2019; Landrigan et al., 2017). Nearly one-third of Canadians live within 100 metres of a major road or 500 metres of a highway, with over 10 per cent of all elementary schools and over 35 per cent of long-term care facilities located within 50 metres of a major road or highway (Brauer et al., 2013). At the same time, a large proportion of Canadians are exposed to transboundary pollution originating from the U.S., particularly in Quebec and Ontario (ECCC, 2017). In dollar terms, the health costs of air pollution are substantial. An estimate by Smith and McDougal (2017), for example, finds that the cost of fine particulate matter and ground-level ozone in Canada was between $26 billion and $48 billion in 2015. A broader analysis by Health Canada (2019) finds that air pollution causes approximately 14,600 premature deaths each year, at a cost of $114 billion—or seven per cent of Canadian GDP.1
Reducing the health risks from air pollution in Canada is closely tied to actions that address climate change. Indeed, one of the most significant opportunities to improve air pollution in the coming decades is capturing the co-benefits from policies primarily aimed at reducing GHGs. Mitigation policies directed at transportation, coal-fired electricity, buildings, and oil and gas extraction have the potential to significantly reduce air pollution. Table 10.1 shows the overlap between sources of GHG emissions and air pollutants.
Canadian governments have already made progress in implementing policies that address both air pollution and GHG emissions. The phase-out of coal-fired electricity in Ontario, for example, reduced SO2 emissions from Ontario’s electricity sector by 99.7 per cent and NOX emissions by 86 per cent (Government of Ontario, 2017). As a result, the number of smog days throughout the province decreased from 53 in 2005 to zero in 2014.2 It also helped reduce electricity sector GHG emissions by 87 per cent. Other notable air pollution/climate policies include efficiency standards for heavy machinery and vehicles and renewable energy policies that displace fossil fuel combustion (ECCC, 2016; 2018b).
Still, more can be done to create cleaner air and reduce GHGs. Notably, particulate matter emissions in Canada increased between 2005 and 2017, driven primarily by an increase in dust emissions (up 44 percent) and emissions from the mining and oil and gas sectors (up 30 per cent and 29 per cent, respectively). Here, the link between climate policy and air pollution is particularly important: not only is particulate matter one of the most harmful types of air pollution for human health, but black carbon—a short-lived climate pollutant—is a key component of fine particulate matter.3
Actions that reduce particulate matter can help drive significant climate and air quality benefits, particularly in urban areas where exposure is highest. Heavy-duty vehicles, for example, represent 15 per cent of Canada’s entire vehicle fleet yet produce 42 per cent of the sector’s total carbon dioxide emissions and 52 per cent of particulate matter emissions (Kodjak, 2015). Policies that encourage greater fuel efficiency or greater uptake of electric and hydrogen-fuel-cell vehicles could make substantial gains in air quality, while also reducing GHG emissions.4
Finally, reducing GHGs does not always improve air quality. Climate policies promoting biomass combustion, for example, can increase PM emissions. At the same time, technologies that scrub out air pollutants from industrial activities can increase energy consumption and therefore increase GHG emissions. Considering the air pollutant implications of GHG policies will be important to fully capturing health benefits in the transition to 2050 (Koornneef et al., 2011).
Although Canada has good data on ambient air quality, governments can improve how they track trends in air pollution and its impacts on human health. Data from Environment and Climate Change Canada’s National Air Pollution Surveillance (NAPS) program (used in Figure 10.1), for example, are only available for 2017 and 2018 (ECCC, 2018a). Ideally, ECCC would make historical data (before 2017) publicly available while also publishing data for years 2019 and beyond. This data would help identify trends over time and areas where air quality poses the largest health risks. Making the NAPS data platform more accessible and user-friendly would also be helpful, as these data are difficult to find and collate.
In addition, expanding monitoring stations can help build a clearer picture of local trends and health risks. The NAPS program is missing data for several major cities (e.g., NO2 and SO2 emissions in Montreal, Quebec City, and Winnipeg) and in Indigenous communities.
Perhaps the biggest data gap is the inability to trace the local sources of air pollution in Canada. ECCC publishes extensive data on air pollution sources through its National Pollutant Release Inventory, but this dataset only covers major industrial (stationary) sources of emissions. It excludes air pollution from commercial and residential buildings, construction, and non-stationary sources, such as transportation, mobile equipment, and wildfires.5 Other datasets from ECCC do track emissions from each of these sectors but they are aggregated at the national level and of little use when examining local trends. Provincial databases (where they exist) also lack information on local pollution sources.
Without better data on the sources of emissions at the local level, determining the causes of air pollution in specific communities is a major challenge. We cannot say with certainty, for example, why NO2 levels in Vancouver are double the levels in other cities, or why Hamilton has extremely high concentrations of SO2 emissions. Better local data would allow policy makers and researchers to identify where air pollution is emitted and prioritize policies that tackle the biggest sources. Such data can also help identify policies that drive the biggest climate and air quality benefits.
More regional airshed modelling should be the ultimate goal of federal, provincial, and local governments. Regional airshed modelling combines data on ambient air quality, pollutant sources, and weather patterns to better understand how different air pollutants mix and move in the atmosphere and how the resulting ambient air pollution affects human health. Models, for example, allow researchers to estimate mortality and morbidity rates from air pollution in each community. These results can then be layered on top of other socio-economic data to see how air pollution affects the most at-risk populations (Indicator #9). Given that airshed modelling is computationally intensive (and expensive), governments could conduct this sort of analysis every five or 10 years to identify patterns and trends.
- Estimate is in 2015 dollars and based on 2015 population counts. It includes annual mortalities associated with three pollutants: PM2.5, NO2, and O3 (Health Canada, 2019). Dollar estimates are likely conservative.
- A similar policy at the national level—implemented after the Ontario coal phase-out—is expected to prevent approximately 1,008 premature deaths and 871 hospital admissions or emergency room visits between 2015 and 2035, a benefit valued at $5 billion (Pembina Institute, 2016). It is also expected to generate $3.4 billion in avoided climate change damage (ECCC, 2018b).
- Black carbon is a component of fine particulate matter and is generated through the incomplete combustion of fossil fuels and biomass (ECCC, 2019c). It is considered a short-lived climate pollutant because it stays in the atmosphere for only a few days or weeks (C2ES, 2020). Black carbon is the third-biggest contributor to global climate change, after methane and carbon dioxide emissions.
- Note that about 13 per cent of total PM2.5 emissions from on-road transportation are generated from brake and tire wear. These emissions would likely be unaffected by fuel efficiency improvements or a shift to EVs (ECCC, 2019a)
- Particulate matter and black carbon emitted from controlled burns are included in ECCC data at the national level, but they do not include emissions from uncontrolled wildfires and do not provide this information at a local level.